Search for the Origins of Life: A Reassessment
Introduction: Putting Together a Perspective
From theories of divine creation such as those illustrated in Genesis to the early beginnings of scientific pursuits as exemplified in notions of ‘spontaneous generation’, our understanding of the origins of life has changed greatly over the past few millennia. With advancements in the fields of biology, molecular biology, biochemistry and many other supporting fields, we are just beginning to see a picture unfolding to that age-old question of how life began. Yet while we may no longer believe in the old accepted fact that life originates from the decaying matter of previous life, and may even distance ourselves from such primitive sciences of yesteryears unless it is to acknowledge our superior understanding of today, there is a vital lesson to be learned in this piece of history—a certain degree of modesty when faced with a question to which we may, in all likelihood, never know the precise answer.
For instance it is at the very limits of our scientific understanding that we pursue the search for the origins of life. In trying to ascertain what could have happened at a point roughly 3.5 billion years ago, we are pressing a question that has been, and still remains to be, beyond our full human comprehension of the world we live in. So in rendering our understanding to explain a ‘how’, we must first inevitably ask a great deal more ‘whys’ in the spirit of science. For a theory which responds to how, for example, simple molecules could come together to form amino acids, is inherently flawed if it is not based in a science to explain why they should even form amino acids in the first place as opposed to other molecules.
In the history of this age-old question, we have more often than not been demonized by certain defects in our human tendencies—to egotism, summary use of incomplete data, and in a subtler but more profound sense, the undermining limitations of our human perception and reasoning. Indeed, few will answer the question currently on the table with a resolute, “It cannot yet be determined”, and those who do will not be published and read about.
The purpose of this paper is to review a few of the major theories on the subject of the origins of life and to discuss not only how they work, but also on what basis they are rooted in our scientific understanding. This paper will obviously not present any new data on the subject that is not already in our present literature, but the hopes are that through a critique of the mechanics of our theories and sciences, we will come to a better understanding of the question at hand.
Updating the Question: Defining the Gap
Our current understanding of the origins of life has progressed from two different directions: one that has moved chronologically forward from the birth of our universe and our planet and one which has attempted to read the story backward through a causal relationship of our present state. In either direction, there is a shared point where our understanding begins to waver and in which lies the crux to the question of the origins of life. Generally speaking, it is the very beginning of life itself; more specifically, it is that bridge between the evolution of simple molecules and the coming together of those molecules to form a replicating and self-organizing system (a tentative description for ‘life’ which we will use at the moment and discuss in more detail later).
So to begin our discussion, let us attempt to define this gap in terms of the two sides which form the break in our understanding. At the first precipice, the beginnings of the early earth, we have already come to the first of many controversial topics. To avoid any lengthy treatment of theories at this point, I will keep my illustration as simple as possible to what can generally be agreed upon for the conditions of the early earth.
Martin Olomucki, scientist and author, believes that at the outset, our universe consisted primarily of hydrogen and helium. Later, through nuclear fusion in stars, about 90 or so heavier elements which were sufficiently stable in the system of the Milky Way were created. On the primitive earth, the four major elements which we will primarily concern ourselves with include hydrogen, carbon, nitrogen and oxygen. These elements are important due their ability to readily form covalent bonds by pooling electrons. Of these four, carbon is especially important because it is capable of accepting or donating four electrons from its outer shell, thus allowing it to form four covalent bonds with four different carbon atoms (or H, O, and N atoms) and giving it the ability to produce a wide range of three-dimensional structures. These four elements also, not inconsequentially, form 99.4% of the human body.1 It is believed that early on these elements were able to come together on the primitive earth to form simple molecules such as hydrogen cyanide (HCN), ammonia (NH3), formaldehyde (CH2O), and water (H2O) (which was plentiful in liquid form). This idea is supported by research conducted by astronomers who have found an abundance of these molecules in the deeps of interstellar space through the use of radio telescopes.2
At this simple setting we will leave our discussion of the early earth. Later discussions of theories of the origins of life will expound what could have happened from this point on.
It is on the second precipice, on the side that contains life, that we know the most of this slowly unfolding story; a remarkable story which would have seemed unimaginable just about 150 years ago. For until then, there would have been no underlying connection between the life of today and the life of long ago. Without a common link, any attempt to ascertain what could have happened billions of years ago in the origins of life question would have proved a troublesome endeavor. For despite our advances in paleontology, individual fossil evidence would mean little without an explanation to unify the workings of life.
So it was in 1859 that Charles Darwin proposed his theory of ‘natural selection’ in his work the Origin of Species. While there are still many opponents to this theory, it has been supported with a good deal of scientific data and so will be treated duly here.
Darwin came to a theory of ‘natural selection’ from three fundamental observations:
- All organisms tend to produce more offspring than can possibly survive.
- Offspring vary among themselves and are not carbon copies of an immutable type.
- At least some of this variation is passed down by inheritance to future generations.3
From these observations, Darwin concluded that if many offspring must die due to the fact that nature’s ecology cannot support the lot of them, and that all individual offspring vary amongst themselves, then on average the survivors will be those that are best suited to changing local environments. Since heredity exists, the offspring of survivors will tend to resemble their successful parents and over time the accumulation of these favorable variants will produce evolutionary change.4
By applying this theory in observing our fossil records from the last few hundreds of millions of years and then looking at the life we are familiar with today, we were able to presume a certain ‘progression’ or degree of development within certain species. Already a link was beginning to emerge. With the understanding of an evolving process in place, we could then theoretically trace the life of today to its very beginning.
Now if we move beyond the simple classification of species, and advance this theory with the recent advancements in molecular biology and biochemistry, a larger picture begins to form. The evidence shows us an underlying and fundamental uniformity at the heart of all life today. This is instanced in the observations that:
- Every creature on earth uses nucleic acids to store its genetic information and they all appear to use the same basic genetic code (although two fairly trivial variations exist).
- This genetic code directs the production of proteins using the same set of 20 amino acids.
- The amino acids are always in the ‘left handed’ form while the sugars in the backbones of genes are always in the ‘right handed’ form.5
- All biological systems use the same currency of energy in the form of ATP.
This evidence could tell us a few different things. Either that the only form of life possible in our universe is the one that follows this chemical makeup as a rule, or, as is popularly theorized, that all life today stems from a single common ancestor. Whatever the reason, whether common ancestor or common chemistry, for the moment we will simply suffice ourselves with the only clear definition of our second precipice—the only mode of life we are familiar with which, at its most rudimentary level, uses nucleic acids to store its genetic code and proteins encoded in that genetic code to carry out different reactions (including replication itself).
So the task now at hand is to figure out how from simple molecules one could come to the degree of complexity exhibited in even the simplest forms of life. This is no easy task, however, as even the modest mycoplasma bacteria, for example, has a DNA strand which consists of 760,000 base pairs—an already quite complex macromolecule which does not even include the organism’s other constituent parts such as its proteins. Even on the lower end of complexity as some viruses such as polyoma with its 5100 base pairs6, the task of answering at least one way that life could have originated will prove quite daunting, if not impossible.
What is Life?: A Search for a Definable Moment
“At a recent meeting in Chicago, a highly distinguished international panel of experts was polled. All considered the experimental production of life in the laboratory imminent, and one maintained that this has already been done-his opinion was not based on a disagreement about the facts but on a definition as to just where, in a continuous sequence, life can be said to begin.”
George Gaylord Simpson7
The earth is estimated to be roughly 4.5 billion years old and fossil evidence shows that microbes existed anywhere from 2.8 to 3.8 billion years ago.8 Somewhere between the time of the birth of the earth and the earliest found records of life, a transformation took place. “Lifeless” matter became organized in a special way and became “alive”. But what is life? And where along in that transformation of matter can we distinguish a “living” organism from its “nonliving” parts?
In raising these questions, we have come to a key point which illustrates, quite profoundly, a subjective nature in our sciences. For although the label of “life” can be easily ascribed to multi-cellular organisms and even quite simply to single-celled ones, the definition becomes quite hazy from there on as we look at entities of a ‘less’ complex nature.
It is here that the boundaries between biology, biochemistry, chemistry and physics begin to thin out, if such boundaries do exist at all. For throughout the years, we have been learning that the fundamental elements which constitute life are in fact basic, inert materials. Enzymes, for example, which allow the reactions necessary for life to exist can, in a different context, be used to catalyze reactions in such externally biotic situations as a laboratory or an industrial plant. So where do we draw the line between the definitions of what is “alive” and what is “not alive” when it comes to inert material and the complex structuring of that material into a whole?
One generally accepted definition offers two criteria necessary for characterizing a living organism: 1.) the faculty of self-organization (metabolism) and 2.) the ability to replicate.9 This definition may seem simple enough, but complications arise when we actually consider the criteria by themselves and together.
Coacervates, for example, which are colloidal droplets held together by electrostatic forces have structures which can allow for a metabolism in the technical sense of the word. Small molecules such as sugars and amino acids can actually penetrate into the membrane of coacervates and can there be converted into another substance which can then diffuse back outside of the membrane. With the addition of an enzyme inside the droplet, such as a phosphorylase, and when placed in a phosphorylated glucose solution, the result will be the accumulation of polysaccharides within and the expulsion of inorganic phosphates without.
As for replication, it has been shown that certain minerals, montmorillonites for example (silicoaluminates with a lamellar structure), can replicate and transfer information. In the presence of water, these minerals exhibit intercrystalline swelling due to the penetration of water molecules between their parallel layers. Above a certain concentration of electrolytes in the water, the crystal disintegrates into independent flakes which can then, given the appropriate ingredients in the culture medium, constitute a center of nucleation that can guide the formation of exact replicas. Such a self-multiplying system can be brought about by freezing-thawing cycles or by alternating periods of rain and drought.
Yet while a form of metabolism can be exhibited in coacervates and a form of replication can be seen in minerals, these two examples are not what we would commonly define as “alive” (although some now might debate this). Some examples, however, which tend to bend the rules of the two required criteria come in the form of certain viruses which are nothing more than a core of DNA surrounded by a protein coat. These viruses, such as the T2 bacteriophage which was pivotal in the further elucidation of the genetic role of DNA, have no ATP-ADP energy system (metabolism) of their own and can therefore not be cultivated in the absence of their host’s cell.10 Much like the many organisms which are obligate intracellular parasites, these viruses can be considered ‘alive’ in a very real sense.
So the definition of life is not as clear-cut as some would think. Along the progression of molecules into the complex structures of the life we are familiar with today, a particular, definable moment for the beginning of life is left open for much debate. Whether we can define it in terms of the origin of a membrane, or a template-replicating nucleic acid, or even to the forces between particles will depend simply on one’s individual sensibilities; the scientific definition for it has not yet been established.
Sparking a Discussion: the Miller-Urey Experiment and Beyond
“Life presumably began in primeval oceans as a result of sequential chemical reactions based on original constituents of atmospheres and oceans, and regulated by principles of physics for self-organizing systems.”
Stephen Jay Gould11
In 1936, the Russian biochemist, A. I. Oparin, became the first to suggest how inert chemicals might link up to form organic chains. He argued that although it was impossible to create life from non-life in our present oxygen-heavy environment (due to the fact that oxygen destroys primitive organic molecules such as amino acids), this might not have been the case in the conditions of the earth billions of years ago. He suggested that there was a reducing atmosphere—with little or no free oxygen—which consisted of such gases as methane, ammonia, water, and Hydrogen. Based on this hypothesis, Stanley L. Miller, in 1953 conducted a simple experiment where he combined Hydrogen (H2), water (H20), Nitrogen (N2), methane (CH4), ammonia (NH3) and carbon dioxide (CO2) in a self-contained apparatus that was supposed to simulate the oceanic and atmospheric conditions of the primitive Earth12 (refer to fig. 1)13. The simulation consisted of heating a miniature, mock ocean of water which forced vapors into an atmosphere of gases where an electrode released constant electrical discharges, which was to simulate lightning and cause the gases to interact; the vapor then was sent through a condenser where it was to condense and fall back into the mock ocean. After letting his apparatus run for a week, Miller found in the water mixture modest yields of glycine and alanine and lower yields of many other amino acids, some among the standard twenty fundamental amino acids.
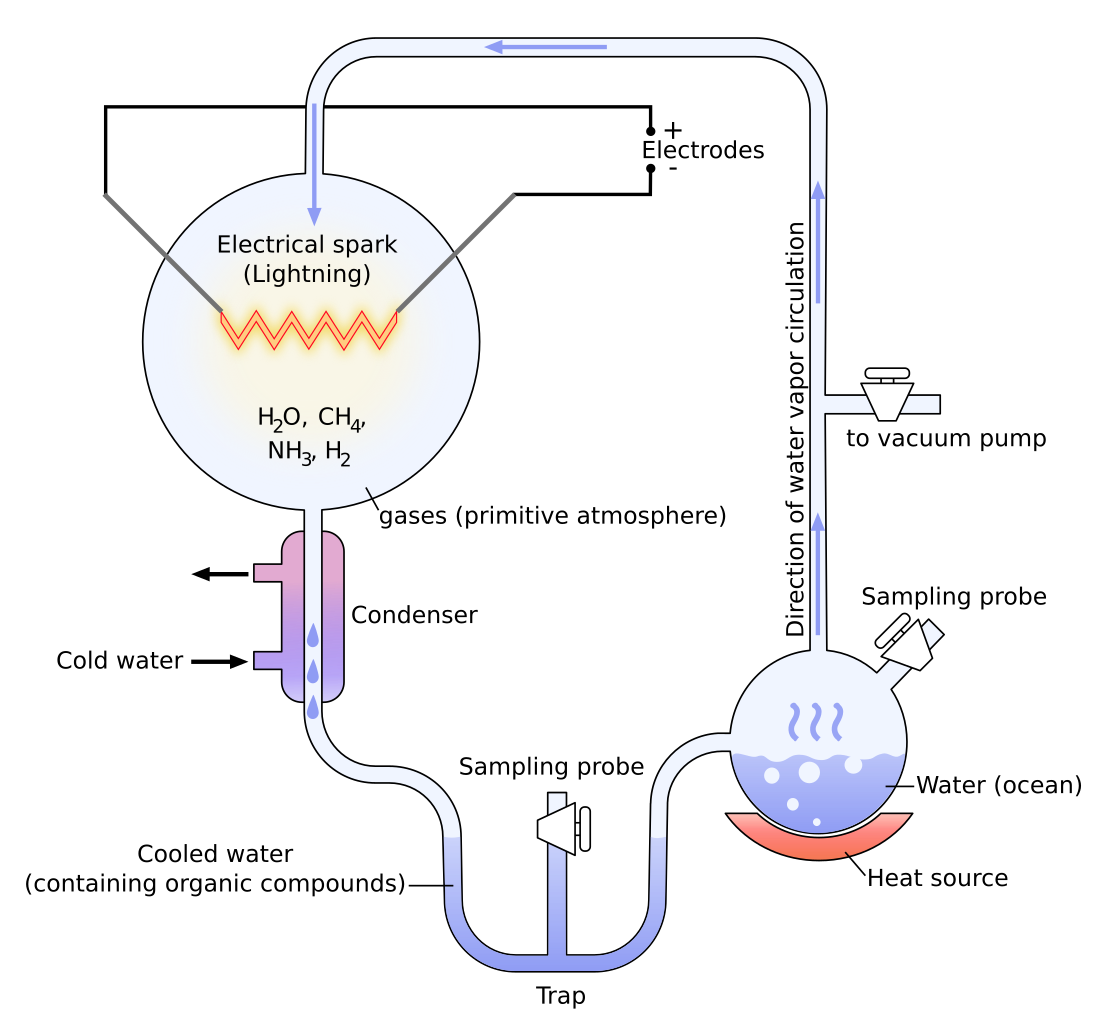
These findings created an immediate uproar in the scientific community as many scientists began to imagine a process for life that could have occurred quite easily and spontaneously. Later, other primitive earth experiments, such as the one conducted by Lowe, Rees, and Markham in 1963 showed the production of the fatty acid building blocks of lipids and the building blocks of RNA and DNA, and another showed the productions of sugars from formaldehyde.14 If we then consider that there are only 30 truly fundamental biomolecules needed to form our type of life, which include twenty amino acids, five nucleobases, two sugars, glycerol, an amino alcohol (choline), and a fatty acid (palmitic acid)15, then it would seem as if the solution to the origin of life question is close at hand.
The basic biochemical theory follows that given the evidence that these precursor monomers could form of their own accord from simpler molecules, they would then collect in the oceans or small bodies of water commonly referred to as the primordial or prebiotic soup. From there, given time, the continuous inflow of energy from sources such as the sun, the inherent characteristics of the matter and the forces which work between them, they would evolve into systems of polymers that through natural selection would evolve into life.
While this is one probable theory for how life could have originated, there are many problems and obstacles along its progression. Firstly, the basis for the primitive earth experiments, the understanding of the conditions of the early earth, are not well founded. There is no real data available to show that the conditions modeled were in fact the conditions of the early earth. Secondly, there is a great deal of criticism nowadays about the manner with which these experiments were, and are, being conducted. Much of this criticism rests on the bias of the experimenters who are accused of finding data in accordance with their own preconceived notions. One such form of this bias is when experimenters, in conducting new primitive earth experiments, select only certain ‘desirable’ molecules from previous experiments, purify them from the other molecules in the solution, and then concentrate them in percentages higher than were normally yielded.16
This practice, which is argued to defeat the time variable of the millions of years in the progression of life, poses many inherent problems in the data collected. This leaves many to believe that the percentage of the molecules of life are greatly exaggerated on the primitive earth. Other factors, not properly represented in the primitive earth experiments, such as the great deal of competition from the other types of molecules formed, ultraviolet light penetrating through the reducing atmosphere and destroying bonds, and just simply the great degree of dilution of molecules in the oceans, offer many obstacles to the biochemical theory of the origins of life.
Replication: Reducing a Series
“The evolution of the genetic machinery is the step for which there are no laboratory models; hence one can speculate endlessly, unfettered by inconvenient facts. The complex genetic apparatus in present-day organisms is so universal that one has few clues as to what the apparatus may have looked like in its most primitive form.”
Richard E. Dickerson17
Clearly, our understanding of the evolution of matter yields much to be desired in an explanation of how life began from simple beginnings. Another area, however, which has been of more help to us in our study has been the mechanism behind the evolution of more complex structures, namely the replication process. It is here that we move away from a strictly sequential explanation of the origins of life and jump again to the side of the evolutionary story which contains life. In assuming Darwin’s theory of natural selection, our understanding follows a simple insight: the fact that life exists on earth now and the assumption that it did not at an earlier time. So if there is a replicating process at the backbone of life, we can trace life to earlier and earlier parent forms and thus come closer to the very origins of life itself.
This line of reasoning, however, can be dangerous if not treated properly. Stephen Jay Gould in his book, Full House, humbled many as to the mechanism behind evolution and renewed criticism over the turn that Darwin’s original theories have taken in the minds of some scientists. In combating a certain human egotism over the direction of nature, he offers the following example: “Equus is the only living genus of horses, and therefore the only modern animal that can serve as an endpoint for a series. If you are committed to depicting the evolution of any living group as a single pathway from an ancestral point to an item of current glory, then I suppose that the story must be told in this conventional way. But when we consider more comprehensive models of evolution, we must call such pictures into question.”18
Systematically, through a statistical look of life on earth, Gould poignantly argues that there is no progressive movement for evolution away from the lowest degree of complexity as exhibited in bacteria. This follows Darwin’s original notions of natural selection which he had originally called “descent with modification”. The word “evolution” did not even appear in Origin of Species and was only accepted when it became part of the mainstream vernacular. Darwin disliked its progressive connotations and even remarked in a letter to the American naturalist, Alpheus Hyatt, “After long reflection, I cannot avoid the conviction that no innate tendency to progressive development exists.”19
But what does this have to do with our discussion on the origins of life? Gould’s argument only brings us from the varied forms of life that exist today to the lowest limit of complexity for a free-living organism, the bacteria. In borrowing, however, from our understanding of how natural selection works on established living forms, we may be able to illuminate the workings which brought us to that life. The important point is that we heed some caution while trying to make sense of evolution and refrain from the short-cuts of unfounded direction. As Darwin once wrote, “Never say higher or lower.”20
So in looking at the heart of life’s progression, the replicating process, what do we know? Essentially, at the root of the evolution of life we are familiar with are the nucleic acids of DNA and/or RNA. It is in this chemical message that we find what may be the best fossil evidence to an earlier time that we have. This is the only message which has persisted and survived over the long term through the making of a countless number of copies—a mode of copying which in our present biology has an error frequency of less than 1 in 100 million nucleotides.21
Now if we can observe this chemical message in all forms of life today and take into consideration other similarities (e.g. an ATP energy system, a common stereo chemistry), then we can make the assumption that all forms of life are descended from one common ancestor. This ancestor, according to Gould and also following a notion for the lowest common denominator, was most probably a bacterium. Therefore, it is this model of simplicity that we will use as an endpoint.
A great deal of trouble, however, arises when we try to suppose what could have happened before this point. For in the movement from life to non-life, we are faced with a perplexing problem that is at the moment an area of heated debate. At the crux of the matter is the fact that while nucleic acids are needed to encode the genetic message for proteins, proteins are needed to translate that message. In the current system, one is of no use without the other. So how could we have come to this highly interdependent relationship in the course of evolving molecules?
A few decades ago the two major opposing camps to this debate were those who believed that life began in an RNA world and those proponents who believed in a protein-first model. The theories advanced by those scientists are still quite controversial, but for a historical perspective I will treat a few of the major theories here.
One of the major theories for a protein-first model of the origins of life comes from Sidney W. Fox, who in the 1950’s conducted experiments on the formation of proteins under primitive Earth conditions. What he found was that amino acids were capable of polymerizing fairly easily to yield polypeptides through reactions which could have been induced by electrical discharges, heat (such as geothermal heat), or through contact with certain types of clays and polyphosphates. These primitive polypeptides, which he referred to as “proteinoids” could reach lengths of up to 150-200 amino acids. He also noted that the proteinoids did not form in completely random arrays dictated by chance, but exhibited a certain degree of selectivity which was to an extent regardless of the concentrations of amino acids present. Fox also found that under certain conditions, the proteinoids agglomerated into “microspheres” with an outer, double layered structure formed by nonpolar groups of amino acids. This layer resembled a natural membrane much like a lipid barrier.22
Fox’s findings do suggest an explanation of how these macromolecules could have ordered themselves in a period directly preceding the emergence of life, but a few major problems still exist, such as how these polypeptides could have replicated to evolve higher-ordered structures. One possible solution can be found in the discovery that certain clays are capable of acting as templates for the formation of these proteins. Still though, how these templates could be transferred to an RNA molecule or a DNA molecule is problematic.
On the other side of this theoretical thinking were those proponents for an RNA-first model for how life began. With an RNA-first model, the problem of replication is answered but without the property of catalysis such as exhibited by proteins, such an ability to replicate would mean little and would not provide for the evolution of a higher-structured order. For certainly, it would be extremely improbable if the reactions needed for life to occur came about only through the random collision of molecules and not through the highly efficient reactions that come about through enzymes. One discovery, however, that has advanced the theory of an RNA-first model for the origins of life was the one that showed that some RNA molecules actually possess certain enzymatic capabilities, such as has been observed in L19 RNA and in the RNA component of ribonuclease P.23
Thomas Cech and Sidney Altman in 1989, furthered such a notion with their theory of early RNAs that had possessed both the ability of replication and catalysis. Such RNA would then later be able to transfer genetic information to the much more stabler DNA molecule through reverse transcriptases (the enzymes that synthesize DNAs with a sequence of bases equivalent to that of a model RNA).24
As for how the synthesis of proteins had come about from this early RNA, a clue is revealed in the self-splicing ability of RNA. In 1987, Alan M. Weiner and Nancy Maizels developed a theory on this in observing the splicing of the 3′-terminal CCA triplet fragment found in tRNA by the ribonucleic component of RNase P. This reaction, which today functions to produce a functional tRNA from a precursor RNA, could have early on been used to release an enzyme RNA. Along with this, Wiener and Maizels theorized that certain variants of RNA-replicases could have acquired a particular activity consisting of joining an amino acid to an RNA. This replicase would have a binding site for the 3′-terminal end of the quasi-tRNA molecule, and when bound to an amino acid could activate it. The resulting activated amino acid could then undergo spontaneous polymerization to yield polypeptides which are short but more functionally useful than peptides with a randomly formed sequence.
In either of these models, the determination of the origins of life is rooted on a mode of causality in an anagenesis form, or an evolutionary style which would resemble a linear chain or ladder. The truth of the matter, however, is that we do not know for sure that life could have even began in the forms we are familiar with. The simplest bacteria that serves as our only firm understanding for an endpoint, may not very well be the beginning in itself but rather part of a middle. One theory which advances this notion is the one made by Graham Cairns-Smith in his book Seven Clues to the Origin of Life..
Cairns-Smith, in attempting to completely rethink the origins of life question, raised the argument that the life of today may actually be descended from an earlier ancestor of an altogether different composition. In confronting the problem of nucleic acids and proteins, he likened the highly interdependent relationship to that of an arch, where a scaffolding would be needed in its construction. Cairns-Smith argued that “our ultimate ancestors’ subsystem were not strongly interdependent (i.e. not arched), and that genetic takeover provided a means of transition to the decidedly ‘arched’ system that we know.”25 Based on the certain characteristics of clays and minerals, he describes a form of crystal life that replicated, made use of its surroundings to its benefit, evolved and then passed on its genetic information to the life of today.
The deduction of this chicken and egg problem of replication just may be the largest step we can take to coming to the origin of life. If, however, the scaffolding for its construction has been lost or the remnants of its early mechanisms became overtaken to be far too negligible in the genetic code of our present biology, then we may never know its answer.
Calling Our Science into Question
Fred Hoyle, a British mathematician, once described the likelihood of the formation of life from inanimate matter as a function of one to a number with 40,000 noughts after it.26 In a statistical sense, the emergence of life may seem like a near impossibility, but only if we consider the process simply in terms of the random collisions between matter. If this were the case, then within the time frame of roughly a billion years, life would probably not have gotten started. Discarding, therefore, any notion of us winning a vast cosmic lottery, there must be other factors at work. Indeed, the existence and emergence of life seems to many scientists to be such a natural occurrence that they believe that life could quite easily exist elsewhere in space.
Why should this be the case though? When scientists look for life in systems whose conditions resemble our own, what are the mechanisms behind this system which would afford the creation of life? So far we have spoken of the ‘how’ in the origins of life, but what is the ‘why’? We may know quite simply that the polymers of life come from simple monomers, or that proteins come from amino acids and nucleic acids from nucleotides, and that these monomers come from even simpler elements, but what is the mechanism behind this progression? Is it truly chance or is there something else at work here?
One clue given to us of this mechanism comes through the work of Prigogine and the laws of nonlinear thermodynamics. According to this theory, spontaneous self-organization becomes a characteristic of ‘dissipative structures’ which produce excess entropy and dissipate it into the environment. In other words, in our system, kept remote from equilibrium by constant flows of energy and matter, dissipation of energy becomes a source for order. This offers a fine insight into the mechanism at work in this progression of structure which brought about life, but what do we really know about it? Denbigh and Denbigh wrote in their book Entropy In Relation to Incomplete Knowledge, “It will be recalled that in 1867 [James] Maxwell corresponded with Tait about the ‘demon’ who could supposedly defeat the Second Law by having a sufficiently detailed perception of the movements and velocities of individual molecules. This led Maxwell to the modern view that the Law is not absolute but is statistical. For instance, in his Theory of Heat (1875) he remarked that the status of the Law would be very different if we had the powers of ‘a being whose faculties are so sharpened that we can follow every molecule in its course.’ Failing such powers, he went on to say, we are compelled to adopt the statistical method of calculation.”27
So what in fact do we really know about our basic physical and chemical sciences aside from a statistical method? When we regard matter in terms of forces of electromagnetism, gravity, and a strong nuclear force, are we fully aware of the mechanisms which drive their interaction? Aside from simply being a metaphysical question, the bearing of these things we do not understand could prove quite profound as we attempt to advance theories on the origins of life.
We therefore need to take fully into account the accuracy of our incomplete sciences. Even in viewing the other of our adopted statistical methods, the one of natural selection, what do we really know about its mechanism? Is there a natural law which presupposes the observation that organisms will vary and that those offspring more fortuitously adapted to an environment will thrive and continue to pass on their traits to the next generation? If we are to agree with Gould and the observation that life retains a predominant and constant bacterial mode that only moves away from a left wall of minimum complexity because that is the only open direction for movement, what happens when we near that left wall and consider the time directly before its construction? Some scientists have furthered a notion of a chemical natural selection, but can we extend natural selection to chemistry? Without a strict replicating process for these molecules, we may be overextending the parameters of the original theory. The survival of the fittest for molecules just may be a consequence of a chemical nature. Martin Olomucki argues that perhaps there is an inherent quality to certain molecular structures that would allow for a feedback mechanism for the agglomeration of ‘useful’ products and the hindrance of ‘useless’ ones.28 This mechanism, however, has not yet been detailed.
This brings us again to these laws of nature which matter followed and found organization in. Cairns-Smith made a valuable contribution to this discussion by opening up the question to factors outside of an organic nature. Inevitably, it will require a multidisciplinary approach to properly attack this question.
To complicate the question even more and play the devil’s advocate, are we even sure that life began on this planet? Instead of just displacing the question, the consequences of this possibility would also displace the system and invalidate our current approaches. Some scientists believe that the time between the birth of this planet and the instance when life first began was not long enough for the entire process to take place. Some believe that life may have been brought here through meteorites or through the spread of germ cells carried along through cosmic radiation. Such a theory would offer a different explanation for the observation that all life seems to have originated from a common ancestor. This possibility cannot be completely ruled out and does not even seem so outlandish if we consider that it could have been intentional. On this subject, Andrew Scott writes, “We can certainly understand the motivation our ancient alien forbears might have had for their act of cosmic fertilization. The desire to perpetuate life is one of the strongest driving forces of all living things, one which could be expected to be retained by natural selection regardless of the types of organisms involved.”29 Barring any sudden, mass human disaster, I would not doubt that at a time near the end of the human race, there would not be a desire to try and spread life out into space. Further, it would not be of a surprise that in this attempt to perpetuate life, the decision to use a bacterial or fungal vessel would not easily win out against the fragile forms of other higher structures, including that of our own.
Taking all of this into consideration, the magnitude of the origins of life question grows exponentially. The question is not only what are we looking for, but on what basis can we rest the proper formulation of the questions. As we continue to wrestle with this age-old question which has plagued our curiosities for a countless number of years, we will have to employ a good deal of discipline, creative thinking, and solid scientific understanding.
Conclusion
Clearly we are still in the early phases of our pursuit for the origins of life. How far exactly we will be able to progress is not known and will most likely test the very limits of our human capacities. But we have come quite a ways and the fruits of our current understanding have given us quite a remarkable and fascinating view of a majesty which, at the risk of sounding overly ardent, finds us as a beautiful part of a greater whole. Nowhere in my research on this subject did I find a scientist who was not immensely affected by the slight grasp we all share of this single question.
1 Olomucki, Martin. The Chemistry of Life. (New York: McGraw-Hill, Inc., 1993). pp. 49-50.
2 Scott, Andrew. The Creation of Life: Past, Future, Alien. (New York: Basil Blockwell, Inc., 1986). p. 56.
3 Gould, Stephen Jay. Full House: The Spread of Excellence from Plato to Darwin. (New York: Harmony Books, 1996) p. 138.
4 Gould. p. 138.
5 Scott. p. 132.
6 Stryer, Lubert. Biochemistry (4th Edition). (New York: W. H. Freeman and Company, 1996). p. 87.
7 Simpson, George Gaylord. “The World into Which Darwin Led Us”. Science: Vol. 131, No. 3405, 1 April 1960, pp.966- 974, p.969. Taken from website http://members.iinet.net.au/~sejones/orignl05.html.
8 Cairns-Smith, A. G. Seven Clues to the Origin of Life. (Cambridge University Press, 1985). p. 5.
9 Olomucki, Martin. The Chemistry of Life. (New York: McGraw-Hill, Inc., 1993). p. 110.
10 Stryer. p. 78.
11 Gould. p. 169.
12 Hitching, Francis. The Neck of the Giraffe. Taken from website http://members.iinet.net.au/~sejones/orignl05.html.
13 Camara, Mark. Taken from website http://fp.bio.utk.edu/camara/Bio102/Lectures/lect09/sld010.htm.
14 Kauffman, Stuart A., The Origins of Order, (New York: Oxford University Press, 1993). p. 288.
15 Olomucki. p.51.
16 Scott. p. 96.
17 Dickerson, Richard E. “Chemical Evolution and the Origin of Life”. Scientific American: Vol. 239, No. 3, September 1978, p.77. Taken from website http://members.iinet.net.au/~sejones/orignl02.html.
18 Gould. p. 61.
19 Gould. p. 137.
20 Gould. p. 137.
21 Stryer. p. 75.
22 Olomucki., pp. 55-56.
23 Stryer, Lubert. Biochemistry 4th Edition. (New York: W.H. Freeman and Company, 1995). p. 232.
24 Olomucki., p. 60.
25 Cairns-Smith. p. 63.
26 Hoyle, Fred. “Hoyle on Evolution”. Nature: Vol. 294, No. 5837, November 12, p. 148. Taken from website http://id-www.ucsb.edu/FSCF/LIBRARY/ORIGINS/QUOTES/OL.html
27 Denbigh, K.G. and Denbigh, J.S.. Entropy In Relation to Incomplete Knowledge. (Cambridge: Cambridge University Press, 1985). pp. 1-2.
28 Olomucki. pp. 75-80.
29 Scott. p. 129.
Good job